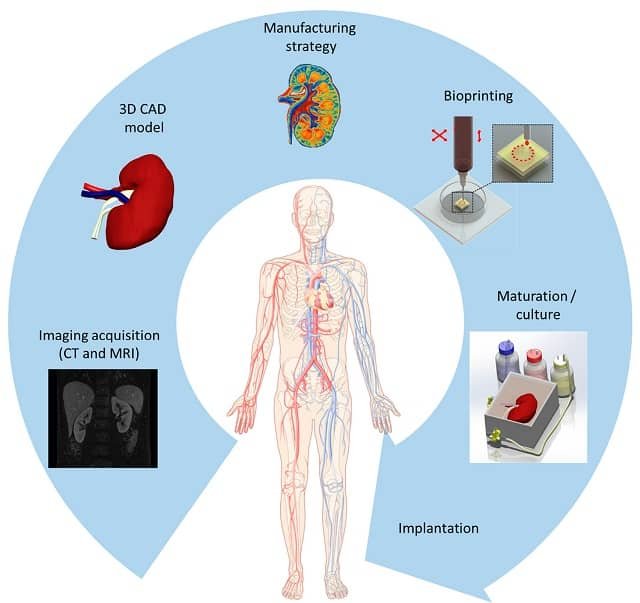
Bioprinting is a revolutionary technology that utilizes 3D printers to create structures of living tissue. This emerging technology has various applications in creating functional tissue constructs to replace injured or diseased tissues (Gungor et al., 2018).
With the ability to manufacture intricate structures layer by layer using bioink and specialized printers, bioprinting promises personalized medicine, organ transplants, and even the regeneration of damaged tissues. Thus, three-dimensional (3D) bioprinting has emerged as a promising approach to designing functional tissues and organs through the precise layer-by-layer positioning of biological materials, living cells, and biochemical components (Fang et al., 2022).
The technology has evolved rapidly in recent years, with advancements in 3D printing techniques, biomaterials, and bioengineering. What was once thought impossible is now within our reach, opening up endless possibilities for healthcare and ultimately, for improving human life.
In this article, we will delve into the various applications of bioprinting, explore its challenges and limitations, and discuss the ethical implications of this transformative technology.
What is 3D bioprinting?
Bioprinting, or 3D bioprinting, is a process similar to traditional 3D printing, but instead of using plastic or metal, it employs bioinks, which are materials containing living cells or other biomaterials. The bioprinter deposits the bioink layer by layer to create a three-dimensional structure that mimics the structure and function of natural tissue.
Sun et al. (2020) and Fu et al. (2022) indicate that 3D bioprinting is a central technology in biofabrication, utilizing cells, proteins, biological compounds, and biomaterials as basic components for 3D-printed biological models, biological systems, and therapeutic products.
Additionally, bioprinting, as highlighted by Singh et al. (2020), employs additive manufacturing (AM) technology that layers bioink with predefined 3D structures developed in computer-aided design (CAD) software.
The history of bioprinting
Bioprinting may seem like a recent technological development, but its roots go back several decades. The concept of 3D printing emerged in the 1980s when Charles Hull built the first 3D printer (Dey and Ozbolat, 2020), laying the groundwork for what would eventually become bioprinting. However, it wasn’t until the early 2000s that scientists began experimenting with the idea of using 3D printers to create living tissues.
The early successful experiments in bioprinting involved printing simple structures like blood vessels and skin. These early breakthroughs paved the way for future advancements in this field.
2D bioprinting vs. 3D bioprinting
Currently, in the academic world, there is discussion of 2D bioprinting, 3D bioprinting, and even 4D bioprinting (which we will discuss later).
2D bioprinting is a simpler and more economical technology, but it is limited to creating simple tissues, whereas 3D bioprinting is a more complex and expensive technology, but it allows for the creation of more complex and realistic tissues.
It is important to note that 3D bioprinting is a rapidly developing field, with new technologies and materials constantly being developed.
Table 01. Comparison between 2D Bioprinting and 3D Bioprinting.
Characteristic | 2D Bioprinting | 3D Bioprinting or 3D Bioprinting |
---|---|---|
Dimension | Creates flat structures. | Creates three-dimensional structures. |
Complexity | Lower complexity, ideal for simple tissues. | Higher complexity, allows for creating more complex and realistic tissues. |
Resolution | Lower resolution in tissue construction. | Greater precision and control over tissue structure. |
Printing Time | Shorter printing time. | Longer printing time, especially for complex structures. |
Materials | Limited variety of available materials. | Greater variety of compatible biomaterials. |
Applications | Primarily used for basic tissue research and development. | Used for a wide range of applications, such as organ creation, implants, and tissue models for research. |
Cost | Lower initial cost. | Higher initial cost due to technology complexity. |
Advantages and Disadvantages
The feasibility and selection of 2D and 3D bioprinting for a specific application will also depend on the availability of cells, biomaterials, and necessary equipment. In the following table, we present a comparison of the advantages and disadvantages of each type of bioprinting.
Table 02. Advantages and Disadvantages of 2D Bioprinting and 3D Bioprinting.
Type of Bioprinting | Advantages | Disadvantages |
---|---|---|
2D Bioprinting | Lower initial cost | Lower complexity |
Shorter printing time | Lower resolution | |
Suitable for simple tissues | Limited variety of available materials | |
Easy to learn and use | Limited to basic tissue research and development applications | |
3D Bioprinting or 3D Bioprinting | Higher complexity | Higher initial cost |
Greater precision and control over tissue structure | Longer printing time | |
Greater variety of compatible biomaterials | More complex to learn and use | |
Used for a wide range of applications |
The choice between 2D and 3D bioprinting will depend on the specific application and the resources available.
The Science Behind Bioprinting
Additive manufacturing (3D printing) is driving significant innovations in many areas such as engineering, manufacturing, art, education, and medicine (Murphy and Atala, 2014), giving rise to 3D bioprinting.
The science behind bioprinting is based on the principles of additive manufacturing, where materials are deposited layer by layer to create a three-dimensional object. In the case of bioprinting, the “ink” used is a combination of living cells and a bioink, which acts as a scaffold to support cell growth and differentiation. The bioink is carefully formulated to mimic the natural environment of cells, providing them with the necessary nutrients and signals for proper development. The printer then precisely deposits the bioink, layer by layer, to create a fully functional tissue or organ. This process is made possible by advances in 3D printing techniques and the development of compatible biomaterials.
Bioprinting is a cutting-edge technology that combines the fields of biology, engineering, and medicine.
Types of Bioprinting
There are three main types of bioprinting:
Extrusion Bioprinting
Extrusion bioprinting is the most commonly used method. Schwab et al. (2020) describe that extrusion bioprinting involves the deposition of filaments from a formulation containing printable cells, which is loaded into a cartridge. The bioink is extruded through a fine nozzle to create three-dimensional structures.
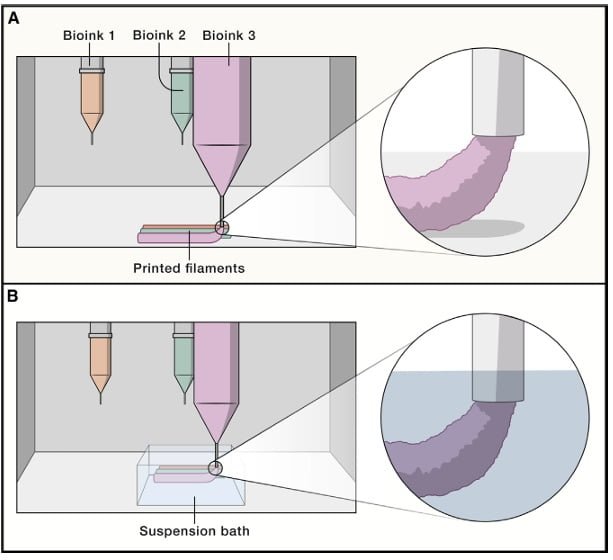
Drop-on-Demand or Inkjet Bioprinting
The drop-on-demand bioprinting method uses a print head similar to that of an inkjet printer to deposit small droplets of bioink. The inkjet technique can generate drops in the picoliter volume range, fire thousands of times in a few seconds, and print without contact (Li et al., 2020).
Laser Bioprinting
Dey and Ozbolat (2020) report that laser-based bioprinting uses laser energy to print 3D structures, such as in stereolithography (SLA), through a principle of photopolymerization; and that it can be used for the precise positioning of cells.
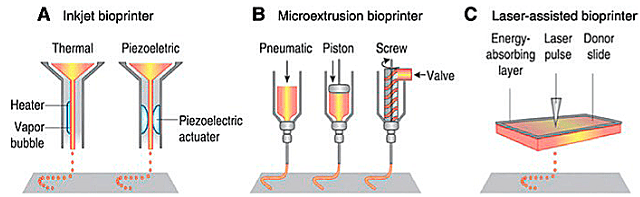
Ink for 3D Bioprinting
According to Schwab et al. (2020), inks for 3D bioprinting can be distinguished into bioinks and biomaterial inks:
Bioinks
Bioinks are materials containing living cells and other components necessary for cell survival. Gungor et al. (2018) highlight that bioinks are a solution of a biomaterial or a mixture of several biomaterials in the form of a hydrogel, which usually encapsulates the desired cell types; and emphasize that an ideal bioink should possess mechanical, rheological, and biological properties appropriate for the target tissues, which are essential to ensure the proper functionality of bioprinted tissues and organs.
Biomaterial Inks
Biomaterial inks are materials used to create support structures for cells. Biomaterial inks do not contain living cells, which impose less strict physicochemical demands and therefore allow for a much wider range of processing parameters (Schwab et al., 2020).
On the other hand, bioprinting companies offer a variety of solutions, from 3D printers and bioinks to software and design services. Some of the leading companies in this field that may inspire you include:
Applications of Bioprinting in Medicine
Bioprinting is an emerging technique that has rapidly evolved (Sun et al., 2020), and has been a powerful tool for creating patterns and accurately placing biological products, including living cells, nucleic acids, drug particles, proteins, and growth factors, to recapitulate the anatomy, biology, and physiology of tissues (Ozbolat et al., 2016).
In turn, Murphy and Atala (2014) highlight that 3D bioprinting is being applied to regenerative medicine to address the need for transplantable tissues and organs. Daly et al. (2021) indicates that there are opportunities to apply it in biology for cellular morphogenesis.
Regenerative Medicine
The applications of bioprinting in medicine are vast and far-reaching. One of the most promising areas is regenerative medicine, where bioprinted tissues and organs can be used to replace damaged or diseased ones. For example, patients in need of an organ transplant often face long waiting lists and the risk of organ rejection. Bioprinting offers a potential solution by allowing the creation of organs on demand, using the patient’s own cells to minimize the risk of rejection. This personalized approach has the potential to save countless lives and revolutionize the field of transplantation.
Drug Development
Another interesting application of bioprinting is in pharmaceutical product development. Traditional drug development often relies on animal testing, which can be time-consuming, costly, and ethically controversial. Bioprinting offers an alternative by allowing researchers to create 3D models of human tissues, which can be used to test the efficacy and safety of new drugs. This approach not only reduces the need for animal testing but also provides a more accurate representation of how drugs will interact with human tissues.
Examples of Bioprinting
Organ Printing
Organs such as hearts, kidneys, and livers have been successfully printed. Bertassoni (2022) reports that advances in the field towards the realistic manufacturing of functional organs have never been so extensive, and his research can be employed as a roadmap on advances and challenges.
Tissue Printing
Tissues such as skin, cartilage, and bone have been successfully printed. Genova et al. (2020) indicates that bone tissue engineering is a branch of regenerative medicine aimed at finding new solutions to treat bone defects, which can be repaired by 3D-printed living tissues; to overcome the limitations of conventional treatment options by improving osteoinduction and osteoconduction.
Challenges and Limitations of Bioprinting
While bioprinting is immensely promising, it is not without challenges and limitations. One of the main obstacles is the complexity of creating functional tissues and organs. The human body is incredibly complex, with different types of cells, structures, and functions. Replicating this complexity in a bioprinted organ is no small feat. Scientists are still working to perfect the techniques and materials needed to create fully functional organs that can seamlessly integrate with the body.
Another challenge is scalability. While successful bioprinting experiments have been conducted on a small scale, scaling up to create larger tissues and organs presents logistical and technical challenges.
Murphy and Atala (2014) indicate that addressing the complexities of bioprinting requires the integration of technologies from the fields of engineering, biomaterial science, cell biology, physics, and medicine.
On the other hand, the bioprinting process is time-consuming and creating larger structures requires overcoming issues such as oxygen and nutrient diffusion. Additionally, the cost of bioprinting is currently high, making it inaccessible for many healthcare institutions and patients.
Sun et al. (2020) summarize the main challenges of bioprinting in:
- Bioinks: the need for a new generation of novel bioinks with multifunctional properties to transport, protect, and better grow cells during and after printing;
- Printing process: better printing processes and printers to deliver cells with high survival capacity and high precision;
- Cross-linking: efficient and effective cross-linking techniques and cross-linkers to maintain the structural integrity and stability of the bioink after printing; and,
- Long-term cell culture: integration with microfluidic devices to provide a simulated long-term physiological environment in which to grow printed models.
Ethical Considerations in Bioprinting
As with any emerging technology, bioprinting raises ethical considerations that must be carefully addressed. One of the main concerns is the potential for misuse or abuse of bioprinting technology. The ability to create living tissues and organs raises questions about creating designer organs or even enhancing human capabilities beyond what is considered natural. Ethical frameworks and regulations will be necessary to ensure the responsible use of bioprinting technology and prevent unethical practices.
Another ethical consideration is the use of animals in bioprinting research. While bioprinting offers the potential to reduce or eliminate the need for animal testing, there are still cases where animals may be involved in the development and validation of bioprinting techniques. Striking a balance between advancing medical knowledge and minimizing harm to animals will be an ongoing ethical challenge in the field of bioprinting.
Bioprinting in the Future: Potential Advances and Possibilities
Looking to the future, bioprinting promises even more remarkable advances. Researchers are exploring the use of bioprinting for complex organs, such as the brain, which present unique challenges due to their intricate structure and functionality.
The ability to bioprint vascular networks is also a focus of ongoing research, as it is crucial for ensuring the survival and functionality of larger bioprinted structures.
In the long term, bioprinting may even enable the creation of personalized and fully functional organs that can seamlessly integrate into the human body.
4D Bioprinting
The Massachusetts Institute of Technology (MIT) invented 4D printing technology, where the fourth dimension refers to time. In this regard, An et al. (2016) define 4D bioprinting as groups of programmable self-assembly, self-folding, or self-accommodation technologies that include three main defining or essential components:
- Human-made programmable design,
- 2D or 3D bioprinting process, and
- Programmable post-printing evolution of bioprinted constructs that could be driven by cells or biomaterials and activated by external signals.
Additionally, Amukarimi and Mozafari (2021) highlight that 4D bioprinting aims to manufacture complex constructs that have the inherent ability to transform their properties in response to internal or external stimuli to repair, regenerate, or replace sick or damaged cells, tissues, and ultimately, organs.
Conclusion
In conclusion, bioprinting is an innovative technology that is changing the future of medicine. With its ability to create living and functional tissues and organs, bioprinting promises personalized medicine, organ transplants, and regeneration of damaged tissues.
While there are still challenges and ethical considerations to address, recent advances in bioprinting technology have brought us closer to harnessing the full potential of this transformative technology. As bioprinting continues to evolve, it has the potential to revolutionize healthcare, improve patient outcomes, and enhance human life in ways we could only imagine in science fiction.
References
Amukarimi, S., & Mozafari, M. (2021). 4D bioprinting of tissues and organs. Bioprinting, 23, e00161.
An, J., Chua, C. K., & Mironov, V. (2016). A perspective on 4D bioprinting. International Journal of Bioprinting, 2(1).
Bertassoni, L. E. (2022). Bioprinting of complex multicellular organs with advanced functionality—Recent progress and challenges ahead. Advanced Materials, 34(3), 2101321.
Daly, A. C., Prendergast, M. E., Hughes, A. J., & Burdick, J. A. (2021). Bioprinting for the Biologist. Cell, 184(1), 18-32.
Dey, M., & Ozbolat, I. T. (2020). 3D bioprinting of cells, tissues and organs. Scientific reports, 10(1), 14023.
Fang, Y., Guo, Y., Liu, T., Xu, R., Mao, S., Mo, X., … & Sun, W. (2022). Advances in 3D bioprinting. Chinese Journal of Mechanical Engineering: Additive Manufacturing Frontiers, 1(1), 100011.
Fu, Z., Ouyang, L., Xu, R., Yang, Y., & Sun, W. (2022). Responsive biomaterials for 3D bioprinting: A review. Materials Today, 52, 112-132.
Genova, T.; Roato, I.; Carossa, M.; Motta, C.; Cavagnetto, D.; Mussano, F. Advances on Bone Substitutes through 3D Bioprinting. Int. J. Mol. Sci. 2020, 21, 7012. https://doi.org/10.3390/ijms21197012
Gungor-Ozkerim, P. S., Inci, I., Zhang, Y. S., Khademhosseini, A., & Dokmeci, M. R. (2018). Bioinks for 3D bioprinting: an overview. Biomaterials science, 6(5), 915-946.
Li, X., Liu, B., Pei, B., Chen, J., Zhou, D., Peng, J., … & Xu, T. (2020). Inkjet bioprinting of biomaterials. Chemical Reviews, 120(19), 10793-10833.
Maresca, J.A.; DeMel, D.C.; Wagner, G.A.; Haase, C.; Geibel, J.P. Three-Dimensional Bioprinting Applications for Bone Tissue Engineering. Cells 2023, 12, 1230. https://doi.org/10.3390/cells12091230
Mota, C., Camarero-Espinosa, S., Baker, M. B., Wieringa, P., & Moroni, L. (2020). Bioprinting: from tissue and organ development to in vitro models. Chemical reviews, 120(19), 10547-10607.
Murphy, S. V., & Atala, A. (2014). 3D bioprinting of tissues and organs. Nature biotechnology, 32(8), 773-785.
Ozbolat, I. T., Peng, W., & Ozbolat, V. (2016). Application areas of 3D bioprinting. Drug discovery today, 21(8), 1257-1271.
Schwab, A., Levato, R., D’Este, M., Piluso, S., Eglin, D., & Malda, J. (2020). Printability and shape fidelity of bioinks in 3D bioprinting. Chemical reviews, 120(19), 11028-11055.
Singh, S., Choudhury, D., Yu, F., Mironov, V., & Naing, M. W. (2020). In situ bioprinting–bioprinting from benchside to bedside?. Acta biomaterialia, 101, 14-25.
Sun, W., Starly, B., Daly, A. C., Burdick, J. A., Groll, J., Skeldon, G., … & Ozbolat, I. T. (2020). The bioprinting roadmap. Biofabrication, 12(2), 022002.